Monday, August 31, 2009
Display problem ? Click HERE
Recommended :
- Subscribe FREE - Chemical Engineering
- Tips on Succession in FREE Subscription
Lower flammable limit (LFL) or Lower Explosive Limit (LEL) is minimum vapor concentration in air which a mixture will burn when an ignition source is present. Upper flammable limit (UFL) or Upper Explosive Limit (UEL) is maximum vapor concentration in air which a mixture will burn when an ignition source is present. Concentration of mixture of vapor in air below LFL/LEL (too lean) or above UFL/UEL (too rich), mixture will not burn even an ignition source is present. Therefore, flammable range or explosive range is concentrations between LFL/UFL and UFL/UEL.
Component LEL & UEL
LFL/LEL and UFL/UEL for some common gases are indicated in table below. Some of the gases are commonly used as fuel in combustion processes.
- Subscribe FREE - Chemical Engineering
- Tips on Succession in FREE Subscription
Component LEL & UEL
LFL/LEL and UFL/UEL for some common gases are indicated in table below. Some of the gases are commonly used as fuel in combustion processes.
Fuel Gas | (LFL/LEL) (%) | (UEL/UFL) (%) |
Acetaldehyde | 4 | 60 |
Acetone | 2.6 | 12.8 |
Acetylene | 2.5 | 81 |
Ammonia | 15 | 28 |
Arsine | 5.1 | 78 |
Benzene | 1.35 | 6.65 |
n-Butane | 1.86 | 8.41 |
iso-Butane | 1.80 | 8.44 |
iso-Butene | 1.8 | 9.0 |
Butylene | 1.98 | 9.65 |
Carbon Disulfide | 1.3 | 50 |
Carbon Monoxide | 12 | 75 |
Cyclohexane | 1.3 | 8 |
Cyclopropane | 2.4 | 10.4 |
Dimethyl Ether | 3.4 | 27 |
Diethyl Ether | 1.9 | 36 |
Ethane | 3 | 12.4 |
Ethylene | 2.75 | 28.6 |
Ethylene Oxide | 3.6 | 100 |
Ethyl Alcohol | 3.3 | 19 |
Ethyl Chloride | 3.8 | 15.4 |
Fuel Oil No.1 | 0.7 | 5 |
Hydrogen | 4 | 75 |
Isobutane | 1.8 | 9.6 |
Isopropyl Alcohol | 2 | 12 |
Gasoline | 1.4 | 7.6 |
Kerosine | 0.7 | 5 |
Methane | 5 | 15 |
Methyl Alcohol | 6.7 | 36 |
Methyl Chloride | 10.7 | 17.4 |
Methyl Ethyl Ketone | 1.8 | 10 |
Naphthalene | 0.9 | 5.9 |
n-Heptane | 1.0 | 6.0 |
n-Hexane | 1.25 | 7.0 |
n-Pentene | 1.65 | 7.7 |
Neopentane | 1.38 | 7.22 |
Neohexane | 1.19 | 7.58 |
n-Octane | 0.95 | 3.20 |
iso-Octane | 0.79 | 5.94 |
n-Pentane | 1.4 | 7.8 |
iso-Pentane | 1.32 | 9.16 |
Propane | 2.1 | 10.1 |
Propylene | 2.0 | 11.1 |
Silane | 1.5 | 98 |
Styrene | 1.1 | 6.1 |
Toluene | 1.27 | 6.75 |
Triptane | 1.08 | 6.69 |
p-Xylene | 1.0 | 6.0 |
Note : The limits indicated are for component and air at 20oC and atmospheric pressure.
Mixture LFL/LEL & UFL/UELA mixture is combustible / flammable within mixture LFL/LEL and UFL/UEL. Common units for both limits is mole (or volume) percent fuel in air [moles fuel/(moles fuel + moles air)]. A mixture LFL/LEL and UFL/UEL limits can be calculated using the equations first proposed by Le Chatelier in 1891 :
Example
A vapor contains of 20 vol% of Methane (C1), 20 vol% of Ethane (C2) and 60 vol% of Propane (C3). Find LEL of this mixture at 20 degC and Atmospheric pressure (101325 kPaA).
LELC1 = 5 vol% at 20 degC & 101.325 kPaA
LELC2 = 3 vol% at 20 degC & 101.325 kPaA
LELC3 = 2.1 vol% at 20 degC & 101.325 kPaA
LELMix = 1 / [ 0.2/5 + 0.2 / 3 + 0.6 / 2.1 ]
LELMix = 2.55 vol% at 20 degC & 101.325 kPaA
Above LEL may be linked to MOC as discussed in "Minimum Oxygen Concentration (MOC) for Flare Purge". Vapor mixture flammability & explosivity at Operating P & T discussed in this post.
Related Topic
- Inert Gas or Fuel Gas For Flare Purge ?
- Nitrogen Purging - What are the factors you need to consider ?
- Personnel Exposure Time For Heat Radiation
- Heat Radiation For Pain & Blistering Threshold
- Fire related topics...
- Flare related topics...
Saturday, August 29, 2009
Display problem ? Click HERE
A fire is formed or a flame can sustains and propagate, three main elements shall present as defined in well known FIRE triangle. There are combustible material (or fuel), oxygen (O2) and heat. See following image. One additional characteristic shall also present is the potential of chain reaction to maintain continuous combustion before any of these three elements is removed. This speed of chain reactions define if a mixture is combustible (slow) or explosive (fast).
Minimum Oxygen Concentration (MOC)
Oxygen is common obtained from atmosphere. Standard air at Mean Sea Level (MSL) contains 20.95 vol% Oxygen (O2), 78.08 vol% Nitrogen (N2), 0.038 vol% Carbin Dioxide (CO2) and others inert gas i.e. Neon, Xenon, etc. Although Oxygen is the major component in generating fire, there is still a minimum oxygen concentration (MOC) required present in combustible mixture so that a fire can be initiated and propagated. Below this limit, a fire will not form.
Following MOC for some hydrocarbon common found in oil and gas plant.
Base on this principle, a flare header is purged with hydrocarbon (i.e. fuel gas ) to evacuate air that ingressed via flare tip and stack in order to ensure quantity of oxygen level in the flare system is always below minimum oxygen concentration (MOC).
MOC For Flare Purge
From above table, you may noticed that MOC for majority of components are equal to or more than 10 vol% except Hydrogen (H2), Acethylene and Carbon Disulfide (CS2). It is recommended MOC of 6 vol% for flare purging design with 4 vol% as design margin. One shall remember plant releasing large amount of Hydrogen shall use lower MOC with margin i.e. 2 vol%. One of the example is Hydrogenation unit in Refinery plant.
Related Topic
Minimum Oxygen Concentration (MOC)
Oxygen is common obtained from atmosphere. Standard air at Mean Sea Level (MSL) contains 20.95 vol% Oxygen (O2), 78.08 vol% Nitrogen (N2), 0.038 vol% Carbin Dioxide (CO2) and others inert gas i.e. Neon, Xenon, etc. Although Oxygen is the major component in generating fire, there is still a minimum oxygen concentration (MOC) required present in combustible mixture so that a fire can be initiated and propagated. Below this limit, a fire will not form.
Following MOC for some hydrocarbon common found in oil and gas plant.
Component | MOC (Vol% O2) |
Hydrogen | 4.0 |
Acetylene | 6.2 |
Methane (C1) | 12.0 |
Ethane (C2) | 11.2 |
Ethylene (C2=) | 9.9 |
Propane (C3) | 11.6 |
Propylene (C3=) | 11.5 |
Butane (C4) | 12.3 |
1-Butene (C4=) | 11.0 |
Pentane (C5) | 11.8 |
Hexane (C6) | 11.8 |
Benzene (Bz) | 11.5 |
Carbon Disulfide | 5.0 |
Base on this principle, a flare header is purged with hydrocarbon (i.e. fuel gas ) to evacuate air that ingressed via flare tip and stack in order to ensure quantity of oxygen level in the flare system is always below minimum oxygen concentration (MOC).
MOC For Flare Purge
From above table, you may noticed that MOC for majority of components are equal to or more than 10 vol% except Hydrogen (H2), Acethylene and Carbon Disulfide (CS2). It is recommended MOC of 6 vol% for flare purging design with 4 vol% as design margin. One shall remember plant releasing large amount of Hydrogen shall use lower MOC with margin i.e. 2 vol%. One of the example is Hydrogenation unit in Refinery plant.
Related Topic
Labels: Fire, Flare, Purging, Safety
Monday, August 24, 2009
Display problem ? Click HERE
The number of large natural gas fields for mankind’s exploitation is reducing as we go into the future. Future natural gas fields are relatively smaller, scattered in various geographical locations and contains higher acidic components. As the world’s energy demand continues, there is an urgent need to develop emerging technologies to monetize small natural gas resources around the globe which could not be economically carried out now.
The emerging technologies have to be safe, economical and more robust than presently available technologies for successful and meaningful gas production. This presentation will share several emerging technologies currently being studied and developed by the oil and gas industry for such purpose.
A talk on "Emerging Technologies to Monetize Small Natural Gas Resources", organized by Chemical Engineering Technical Division, IEM has been scheduled.
Date : 10 October 2009 (Saturday)
Time : 9.00 am – 11.00 am (Refreshment would be served at 11.00 am)
Venue : C&S Lecture Room, 2nd Floor, Wisma IEM, Petaling Jaya
Time : 9.00 am – 11.00 am (Refreshment would be served at 11.00 am)
Venue : C&S Lecture Room, 2nd Floor, Wisma IEM, Petaling Jaya
Related Post
Saturday, August 22, 2009
Display problem ? Click HERE
Recommended :
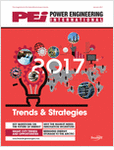
Flare is commonly installed in oil and gas process plant to burn hydrocarbon and/or toxic gas to avoid formation of combustible mixture, to minimize green house effect (GHE), to minimize health hazards to personnel on site, etc. There are several earlier posts related to Flare :
- Quick Estimate Flare Stack Diameter
- Estimate Subsonic Flare Tip Pressure Drop With Graph Derived Correlation
- Quick Estimate Flare Tip Pressure Drop
- Flare Tip Type Quick Selection Chart
- Guideline on Quick Determination of Flare Stack Support Type
- Flare Smokeless Ringlemann Chart
- FLARE combustion efficiency
- Quick Estimate Ground Level Unburnt Flammable Gas For Vent Pipe or Flame-out Flare Stack
Flare collection header is normally "NO flow" as most (if not all) devices connected to flare header are non-discharging fluid into flare system. Among all are pressure relief valve (PRV), blowdown valve (BDV), overpressure dump valve (PCV), etc. All these devices are kept as close position during normal plant operation and will only open in the event of overpressure, emergency situation i.e. fire, runaway reaction, plant shutdown/blowdown for maintenance.
On the flare tip end, it is open to atmosphere. it is very likely that atmosphere air contain oxygen ingress and stay into flare collection header. PRV/BDV/PCV passing and open on demand will discharge large quantity of hydrocarbon gas into flare collector header filled with air and create combustible mixture, as this combustible mixture travel along flare header and reach flare tip which equipped with flare pilot, combustible mixture will be ignited and potentially created flash back to the flare header and flare knock-out drum (KOD). Subject to flare header capacity and mechanical integrity, large flash back lead to severe internal pressure act on the piping & vessel and vapor wave results severe vibration and movement of structure, this potential lead to catastrophe failure of flare collection system. Therefore a flare header is sweep or purge with fuel gas or inert gas i.e. Nitrogen.
Advantages using inert gas compare to fuel gas as purge gas
i) Environment & Green House Effect (GHE)
IG : Inert gas has NO impact to environment
FG : Burn fuel gas in atmosphere generate Carbon Dioxide (CO2) which contributes to increase of Co2 content in atmosphere and increases Green House Effect (GHE)
ii) Burn back damage flare tip - reduce life span of flare tip
IG : Inert gas do not burn. NO burn back and potential damage of flare tip.
FG : Potential FG burn back damage flare and shorten flare tip life span.
iii) High OPEX avoid Burn back
IG : NO burn back. Minimum purge rate and low OPEX.
FG : Potential burn back lead to high purge rate (potential 10 times higher than purge rate of IG) and high OPEX
iv) Visible Flame
IG : Inert gas do not burn. No flame present.
FG : FG continuous burn and continuous visible flare at flare tip. Potential create uneasy situation in environment sensitive area.
v) Smoke Flaring
IG : Inert gas do not burn. No smoke flaring issue.
FG : Burning heavy FG lead to smoke flaring. Potential create uneasy situation in environment sensitive area. Increase likelihood of unburnt component and impact on environment.
vi) Steam injection for smokeless flaring
IG : Inert gas do not burn. No smoke flaring issue.
FG : Burning heavy FG lead to smoke flaring. Steam injection to reduce/eliminate smoke flaring. This increases CAPEX (additional steam injection facilities) and OPEX (steam loss).
vii) Radiation
IG : Inert gas do not burn. No additional radiation.
FG : Fuel gas burn lead to increase of radiation level (on top of solar radiation) to personnel working near flare stack.
Disadvantages using inert gas compare to fuel gas as purge gas
a) Fuel Gas Readily Available in Plant
IG : Required Nitrogen generator or use of Liquid Nitrogen and evaporator. Additional CAPEX and OPEX.
FG : Fuel gas readily available in plant. Minimum CAPEX and OPEX. Some plant generate hydrocarbon gas which shall be disposed off. This gas is readily serve as purge gas and inccur NO cost.
b) Inert Gas Cloud
IG : Flare system purge with inert gas, entire flare system is filled with IG gas (which potential heavier than air). Once any PSV/BDV open and release large amount of gas into flare header, it will "push" IG release through the flare tip. Heavy IG (compare to air) will sink create a IG gas cloud near plant. This is potential fatal thread (suffocation) to personnel on site.
FG : Continuous flaring lead to no or nearly no potential of gas present in atmosphere
c) Unburnt hydrocarbon gas emission
IG : PRV/BDV/PCV leak or passing lead to low heating value mixure (less than 200 btu/ft3) which is non-combustible. Release of hydrocarbon gas into atmosphere directly has more GHE impact than burning it. For example 1 mol of methane create 1 mol of CO2 if it is burnt. 1 mol of methane create 20-21 mol of equivalent CO2 if it unburnt.
FG : Continuous flaring lead to no or nearly no unburnt gas in atmosphere
d) Combustible Cloud lead to Instant Ignition
IG : Slowly hydrocarbon gas emission to atmosphere and built-up of combustible mixture in the plant, once the heating value for auto-ignition is reached, the combustible mixture potentially ignited. Its impact is just like a explosion and potential thread to personnel and surrounding facilities.
FG : Continuous flaring lead to no or nearly no unburnt gas in atmosphere
Concluding remark
Inert gas purging is normally understood as clean, low CAPEX, low OPEX, etc and regards as most likely candidate for flare purging. However, the associated SAFETY related issue may needs additional attention and focus. All...use inert gas wisely...
Related Topic
On the flare tip end, it is open to atmosphere. it is very likely that atmosphere air contain oxygen ingress and stay into flare collection header. PRV/BDV/PCV passing and open on demand will discharge large quantity of hydrocarbon gas into flare collector header filled with air and create combustible mixture, as this combustible mixture travel along flare header and reach flare tip which equipped with flare pilot, combustible mixture will be ignited and potentially created flash back to the flare header and flare knock-out drum (KOD). Subject to flare header capacity and mechanical integrity, large flash back lead to severe internal pressure act on the piping & vessel and vapor wave results severe vibration and movement of structure, this potential lead to catastrophe failure of flare collection system. Therefore a flare header is sweep or purge with fuel gas or inert gas i.e. Nitrogen.
Advantages using inert gas compare to fuel gas as purge gas
i) Environment & Green House Effect (GHE)
IG : Inert gas has NO impact to environment
FG : Burn fuel gas in atmosphere generate Carbon Dioxide (CO2) which contributes to increase of Co2 content in atmosphere and increases Green House Effect (GHE)
ii) Burn back damage flare tip - reduce life span of flare tip
IG : Inert gas do not burn. NO burn back and potential damage of flare tip.
FG : Potential FG burn back damage flare and shorten flare tip life span.
iii) High OPEX avoid Burn back
IG : NO burn back. Minimum purge rate and low OPEX.
FG : Potential burn back lead to high purge rate (potential 10 times higher than purge rate of IG) and high OPEX
iv) Visible Flame
IG : Inert gas do not burn. No flame present.
FG : FG continuous burn and continuous visible flare at flare tip. Potential create uneasy situation in environment sensitive area.
v) Smoke Flaring
IG : Inert gas do not burn. No smoke flaring issue.
FG : Burning heavy FG lead to smoke flaring. Potential create uneasy situation in environment sensitive area. Increase likelihood of unburnt component and impact on environment.
vi) Steam injection for smokeless flaring
IG : Inert gas do not burn. No smoke flaring issue.
FG : Burning heavy FG lead to smoke flaring. Steam injection to reduce/eliminate smoke flaring. This increases CAPEX (additional steam injection facilities) and OPEX (steam loss).
vii) Radiation
IG : Inert gas do not burn. No additional radiation.
FG : Fuel gas burn lead to increase of radiation level (on top of solar radiation) to personnel working near flare stack.
Disadvantages using inert gas compare to fuel gas as purge gas
a) Fuel Gas Readily Available in Plant
IG : Required Nitrogen generator or use of Liquid Nitrogen and evaporator. Additional CAPEX and OPEX.
FG : Fuel gas readily available in plant. Minimum CAPEX and OPEX. Some plant generate hydrocarbon gas which shall be disposed off. This gas is readily serve as purge gas and inccur NO cost.
b) Inert Gas Cloud
IG : Flare system purge with inert gas, entire flare system is filled with IG gas (which potential heavier than air). Once any PSV/BDV open and release large amount of gas into flare header, it will "push" IG release through the flare tip. Heavy IG (compare to air) will sink create a IG gas cloud near plant. This is potential fatal thread (suffocation) to personnel on site.
FG : Continuous flaring lead to no or nearly no potential of gas present in atmosphere
c) Unburnt hydrocarbon gas emission
IG : PRV/BDV/PCV leak or passing lead to low heating value mixure (less than 200 btu/ft3) which is non-combustible. Release of hydrocarbon gas into atmosphere directly has more GHE impact than burning it. For example 1 mol of methane create 1 mol of CO2 if it is burnt. 1 mol of methane create 20-21 mol of equivalent CO2 if it unburnt.
FG : Continuous flaring lead to no or nearly no unburnt gas in atmosphere
d) Combustible Cloud lead to Instant Ignition
IG : Slowly hydrocarbon gas emission to atmosphere and built-up of combustible mixture in the plant, once the heating value for auto-ignition is reached, the combustible mixture potentially ignited. Its impact is just like a explosion and potential thread to personnel and surrounding facilities.
FG : Continuous flaring lead to no or nearly no unburnt gas in atmosphere
Concluding remark
Inert gas purging is normally understood as clean, low CAPEX, low OPEX, etc and regards as most likely candidate for flare purging. However, the associated SAFETY related issue may needs additional attention and focus. All...use inert gas wisely...
Related Topic
- Flare related topics...
- CO2 related topics...
- Personnel Exposure Time For Heat Radiation
- Heat Radiation For Pain & Blistering Threshold
- Simplified Equation for Wind Speed Estimation At Different Height
- Estimate Wind Speed At Flare Tip At Different Height
- Assess AIV with "D/t-method" with Polynomial PWL Limit Line
- Model Fix Pressure Drop Device in FLARENET
Labels: CO2, Environment, Fire, Flare
Thursday, August 20, 2009
Display problem ? Click HERE
Recommended :
- Subscribe FREE - Chemical Engineering
- Tips on Succession in FREE Subscription
If you have successfully subscribed to FREE Chemical Engineering (CE) magazine, you should have received a note from the publisher requesting you to participate a survey in order to help in making Chemical Engineering an even more interesting and informative publication for readers . The survey contain 21 simple questions and should only take a few minutes (less than 3 minutes) to complete.
Chemical & Process Technology webblog member (FREE CE subscriber) is encouraged to participate this survey.
- Subscribe FREE - Chemical Engineering
- Tips on Succession in FREE Subscription
Chemical & Process Technology webblog member (FREE CE subscriber) is encouraged to participate this survey.
Why ?
Reason being
i) to provide your inputs to make Chemical Engineering and so that information can be delivered in effective manners
ii) to serve your commitment to Chemical Engineering Magazine as a free magazine subscriber
iii) to increase your "points" so that you qualify again for free Chemical Engineering during next renewal
What to do ?
You as valid FREE CE subscriber, you will receive a message similar to above image. Just click the "Start the survey" button and it will direct you to complete the 3 minutes survey.
FREE Chemical Engineering Subscription Released every month (almost) but limited ! Quick act before they are fully subscribed. Before proceed, get some tips in "Tips on Succession in FREE Subscription". If FREE CE is temporarily unavailable, please try again beginning of the month (tips). Click here to qualify your FREE Chemical Engineering !
Related Post
Labels: E-Doc, Education, Learning
Saturday, August 15, 2009
Display problem ? Click HERE
Recommended :
- Subscribe FREE - Chemical Processing
- Tips on Succession in FREE Subscription
Plant in country experience subzero ambient temperature, one of the common natural phenomenon is increased heat loss from piping to ambient lead to low temperature fluid. Low temperature fluid will results fluid characteristic change i.e. low reactivity, increased viscosity, promote freezing, crystallization, etc and subsequently lead to several problem such as low productivity, high pressure drop, blockage, scaling, fouling, etc. It is important to design plant system to minimize heat loss to ambient.
Water system at subzero may freeze and lead to blockage and potentially cause pipe damage. One of the effective and non-costly method is utilize low conductivity of soil to reduce heat to ambient by burying water pipe. This may involve a typical heat loss calculation.
Above image is a typical buried pipe in soil.
Heat loss,
with Shape factor S
where
Q = heat loss from pipe, W
S = Shape factor, m
Km = Average soil conductivity, W/m-K
T1 = Pipe skin temperature, degC (or K)
T2 = Soil surface temperature, degC (or K)
PI = 3.141592654
L = Buried pipe length, m
z = Distance between the ground surface and the center of the buried pipe, m
D = Outside diameter, m
Above equations are applicable when L is large compare to D and z is larger than 1.5D.
Example
Km = 0.9 W/m-K
T1 = 80 degC
T2 = 10 degC
PI = 3.141592654
L = 30 m
z = o.5 m
D = 0.1 m
L is much larger than D and z = 0.5 larger than 1.5(0.1) = 0.15
Above equations may be used.
Shape factor,
Heat loss,
- Subscribe FREE - Chemical Processing
- Tips on Succession in FREE Subscription
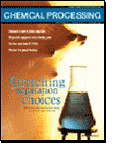
Water system at subzero may freeze and lead to blockage and potentially cause pipe damage. One of the effective and non-costly method is utilize low conductivity of soil to reduce heat to ambient by burying water pipe. This may involve a typical heat loss calculation.
Above image is a typical buried pipe in soil.
Heat loss,
Q = S x Km x (T1 - T2)
with Shape factor S
S = 2 x PI x L / Ln [ 4 z / D ]
where
Q = heat loss from pipe, W
S = Shape factor, m
Km = Average soil conductivity, W/m-K
T1 = Pipe skin temperature, degC (or K)
T2 = Soil surface temperature, degC (or K)
PI = 3.141592654
L = Buried pipe length, m
z = Distance between the ground surface and the center of the buried pipe, m
D = Outside diameter, m
Above equations are applicable when L is large compare to D and z is larger than 1.5D.
Example
A 30m long 100mm diameter hot water pipe of district heating system is buried in the soil 500mm below the ground surface (from pipe center line). Ground surface exposing wind chilling is 10 degC whilst pipe pipe skin temperature is expected to be 80 degC. Determine heat loss from pipe if soil average conductivity is about 0.9 W/m-K.
Km = 0.9 W/m-K
T1 = 80 degC
T2 = 10 degC
PI = 3.141592654
L = 30 m
z = o.5 m
D = 0.1 m
L is much larger than D and z = 0.5 larger than 1.5(0.1) = 0.15
Above equations may be used.
Shape factor,
S = 2 x PI x L / Ln [ 4 z / D ]
S = 2 x PI x 30 / Ln [ 4 x 0.5 / o.1 ]
S = 62.9 m
S = 2 x PI x 30 / Ln [ 4 x 0.5 / o.1 ]
S = 62.9 m
Heat loss,
Q = S x Km x (T1 - T2)
Q = 62.9 x 0.9 x (80 - 10)
Q = 3963 W
Ref : Section 3-7, Heat Transfer A Practical Approach by Yunus A. Cengel, 2nd Ed.
Above equations have been programmed by Ankur, a experience Chemical Engineer, share with readers of Chemical and Process Technology. You may download here.
Thanks to Ankur
Download
*If you have any useful program and would like to share within our community, please send to me.
Related Topics
Q = 62.9 x 0.9 x (80 - 10)
Q = 3963 W
Ref : Section 3-7, Heat Transfer A Practical Approach by Yunus A. Cengel, 2nd Ed.
**********************************
Above equations have been programmed by Ankur, a experience Chemical Engineer, share with readers of Chemical and Process Technology. You may download here.
Thanks to Ankur
Download
*If you have any useful program and would like to share within our community, please send to me.
Related Topics
- HEAT TRANSFER
- Therminol Reference Disk - Assist you to Optimize your Heat Transfer System
- FAYF - Heat Transfer - Useful Heat Transfer Equation
- Heat Transfer - Internal and External Flow
- FREE E-book........A Heat Transfer Textbook
- Few Tips on Energy Efficient & Recovery
- Heat Transfer Coefficient For Air-Cooled Heat Exchangers
- COLLECTION of Typical Overall Heat Transfer Coefficient (U-factor)
Labels: Heat Transfer
Wednesday, August 12, 2009
FREE Chemical Engineering Digital Issue for August 2009 has been released !
Chemical Engineering has just released FREE August 2009 issue. If you are subscriber of Chemical Engineering, you should have received similar notification.
***********************
Interesting articles for this month :
FAYF - Adsorption
This one-page guide explains various adsorbent properties and provides the corresponding
equations
Viscosity: The Basics
An important concept, sometimes forgotten, is that viscosity is not a single-point measurement.
Basic concepts and terminology are reviewed and techniques for quantifying viscosity are addressed
REACH: Looking Beyond Pre-registration
The next steps to ensuring compliance are significantly more complex
Keeping Cooling Water Clean
Proper monitoring and control of water chemistry is essential
Today’s SIS: Integrated Yet Independent
Bringing process control and instrumented safety systems together while still keeping them separate provides benefits beyond risk reduction
Monitoring Air Emissions
New requirements are spurring strong demand in air pollution monitoring
***********************
TIPS
If you are subscriber, you may access previous digital releases. Learn more in "How to Access Previous Chemical Engineering Digital Issue".
If you yet to be subscriber of Chemical Engineering, requested your FREE subscription via this link (click HERE). Prior to fill-up the form, read "Tips on Succession in FREE Subscription".
Related Post
- How to Access Previous Chemical Engineering Digital Issue
- Tips on Succession in FREE Subscription
- 3 Most Important & FREE Magazines That I Read...
- Non - Technical Quick References for a Chemical & Process Engineers
- More You Share More You Learn
- Knowledge is Own by Everyone but Not Someone
Labels: E-Doc, Education, Learning
Sunday, August 9, 2009
Recommended :
- Subscribe FREE - Chemical Engineering
- Tips on Succession in FREE Subscription
Earlier post "Heat Radiation For Pain & Blistering Threshold" and "Personnel Exposure Time For Heat Radiation" have discussed the heat radiation level lead to plant personnel pain and blistering threshold within an exposure time. Heat radiation design criteria for personnel exposure has been proposed for continuous and emergency exposure. Flare radiation run using software i.e. FLARESIM or calculation using Brzustowski and Sommer method, heat radiation level will be estimated and presented. For equipment, instrument, piping, painting, steel structure, etc expose to flare radiation will experienced increase temperature.
How shall a heat radiation level relates to temperature ?
- Subscribe FREE - Chemical Engineering
- Tips on Succession in FREE Subscription
How shall a heat radiation level relates to temperature ?
Heat level (Et) at recepting location can be related to the sum of radiation heat (Er) plus convection heat (Ec).
Et = Er + Ec
For unit area (m2),
qt = qr + qc
where
qt = total heat flux at receptor (kW/m2)
qr = radiation heat flux at receptor (kW/m2)
qc = convection heat flux at receptor (kW/m2)
Radiation heat flux,
qr = sigma x (T4 - Ta4)
Convection heat flux
qc = (h / e) x (T - Ta)
where
sigma = stefan-Boltzman constant = 5.67 e -11 (kW/m2K)
h = convective heat transfer co-efficient = 0.007 (kW/m2K) at zero wind speed
e = Emissivity (0.8-0.9 for copper or rusted CS pipe, 0.1-0.2 for polished SS pipe)
T = Surface temperature (K)
Ta = Ambient temperature (K)
Therefore
qt = sigma x (T4 - Ta4) + (h / e) x (T - Ta)
If a heat radiation level is known at particular location, the corresponding temperature may be estimated using above equation.
Related Topic
- Personnel Exposure Time For Heat Radiation
- Heat Radiation For Pain & Blistering Threshold
- Simplified Equation for Wind Speed Estimation At Different Height
- Estimate Wind Speed At Flare Tip At Different Height
- Assess AIV with "D/t-method" with Polynomial PWL Limit Line
- Model Fix Pressure Device in FLARENET
- Several Criteria and Constraints for Flare Network - Process
- Several Criteria and Constraints for Flare Network - Piping
Labels: Environment, Flare
Monday, August 3, 2009
Display problem ? Click HERE
Recommended :
Subscribe FREE - Chemical Processing
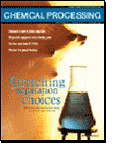
Earlier post "Heat Radiation For Pain & Blistering Threshold" has briefing discussed about the exposure time for heat radiation level which lead pain and blister threshold. Equations have been proposed in order to link between exposure time for dedicated heat radiation. Example, with heat radiation of 6.3 kW/m2 (2 000 Btu/h·ft2), the pain threshold is reached in 8 s and blistering occurs in 20 s.
Maximum allowable personnel exposure time to particular heat radiation level proposed in API RP 521 (previous revision - 1997) and API Std 521 (latest revision - May 2008) are marginally different. Detail list out as follow
Above listing shows that latest API Std 521 has more stringent requirement compare to previous revision (1997), specifically for 6.31 and 4.73 kW/m2. The maximum allowable exposure time limit have been reduced. Continuous exposure limit remain unchanged.
Emergency Exposure
Maximum allowable exposure heat radiation of 6.31 and 4.73 kW/m2 (during emergency scenario) have been used as criteria in determining sterile area, an area around flare stack which no personnel shall be around without any personnel protective apparel. For conservative design and/or expect potential increase in flaring rate in future, a limit of 4.73 kW/m2 may be used. For common design, heat radiation limit of 6.31 kW/m2 is widely used. In general, maximum allowable heat radiation of 9.46 kW/m2 will be limited at the flare stack base where personnel may access to sterile area with proper personnel protective apparel.
Continuous Exposure
For continuous exposure, operator is assumed wearing appropriate clothing in a plant. Heat radiation at any location shall be limited to 1.58 kW/m2.
Related Topic
Subscribe FREE - Chemical Processing
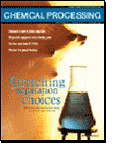
Earlier post "Heat Radiation For Pain & Blistering Threshold" has briefing discussed about the exposure time for heat radiation level which lead pain and blister threshold. Equations have been proposed in order to link between exposure time for dedicated heat radiation. Example, with heat radiation of 6.3 kW/m2 (2 000 Btu/h·ft2), the pain threshold is reached in 8 s and blistering occurs in 20 s.
Maximum allowable personnel exposure time to particular heat radiation level proposed in API RP 521 (previous revision - 1997) and API Std 521 (latest revision - May 2008) are marginally different. Detail list out as follow
Heat Radiation (kw/m2) | API Rp 521 (March 1997) | API Std 521 (May 2008) |
9.45 | Exposure must be limited to a few (approx. six) seconds, sufficient for escape only. May consider tower or structure provide some degree of shielding. | Required urgent emergency action. Radiation shielding and/or special protective apparel (e.g. a fire approach suit) required |
6.31 | Emergency actions lasting up to 1 minutes without shielding but with appropriate clothing. | Emergency actions lasting up to 30s without shielding but with appropriate clothing. |
4.73 | Emergency actions lasting up to several minutes without shielding but with appropriate clothing. | Emergency actions lasting 2 min to 3 min without shielding but with appropriate clothing. |
1.58 | personnel with appropriate clothing can be continuously exposed | personnel with appropriate clothing can be continuously exposed |
Above listing shows that latest API Std 521 has more stringent requirement compare to previous revision (1997), specifically for 6.31 and 4.73 kW/m2. The maximum allowable exposure time limit have been reduced. Continuous exposure limit remain unchanged.
Emergency Exposure
Maximum allowable exposure heat radiation of 6.31 and 4.73 kW/m2 (during emergency scenario) have been used as criteria in determining sterile area, an area around flare stack which no personnel shall be around without any personnel protective apparel. For conservative design and/or expect potential increase in flaring rate in future, a limit of 4.73 kW/m2 may be used. For common design, heat radiation limit of 6.31 kW/m2 is widely used. In general, maximum allowable heat radiation of 9.46 kW/m2 will be limited at the flare stack base where personnel may access to sterile area with proper personnel protective apparel.
Continuous Exposure
For continuous exposure, operator is assumed wearing appropriate clothing in a plant. Heat radiation at any location shall be limited to 1.58 kW/m2.
Related Topic
- Heat Radiation For Pain & Blistering Threshold
- Simplified Equation for Wind Speed Estimation At Different Height
- Estimate Wind Speed At Flare Tip At Different Height
- Assess AIV with "D/t-method" with Polynomial PWL Limit Line
- Model Fix Pressure Device in FLARENET
- Several Criteria and Constraints for Flare Network - Process
- Several Criteria and Constraints for Flare Network - Piping
- Provide More than One Flare KOD in SERIES
Labels: Environment, Flare